What are the advantages of in-depth thermal analysis? A potent method for understanding complex systems.
The term "deephot" is likely a portmanteau combining "deep" and "hot." This implies an in-depth analysis of thermal properties, often at high temperatures. It could describe a specialized research methodology focusing on the relationship between temperature and material properties. For example, a study investigating the thermal conductivity of metals at elevated temperatures could be described using this term.
Such analysis is important in various fields, including materials science, engineering, and astrophysics. Understanding how materials behave under extreme thermal conditions is crucial for designing more efficient engines, developing advanced materials with specific thermal properties, or even modeling the interiors of stars. High-temperature experiments provide invaluable insight into reaction kinetics, phase transformations, and mechanical strength, ultimately leading to innovations in a range of industries. Historical scientific advancements in thermal analysis have directly paved the way for many modern technologies.
The next sections will explore specific applications of thermal analysis in more detail, focusing on how deep thermal investigations are used to advance various technologies.
Deep Thermal Analysis
In-depth thermal analysis, often involving high temperatures, is crucial for understanding material behavior and advancing technology. This analysis unveils critical information across various fields.
- High temperatures
- Material properties
- Reaction kinetics
- Phase transformations
- Thermal conductivity
- Mechanical strength
- Advanced materials
These aspects interconnectedly contribute to the advancement of various technologies. High-temperature testing reveals how materials behave under extreme conditions. Understanding reaction kinetics helps optimize processes. Examining phase transformations assists in developing materials with tailored properties. Measuring thermal conductivity is essential for efficient heat transfer. High-temperature trials illuminate the durability and strength of materials. This process allows the creation of new advanced materials for industries like aerospace or energy. The understanding of these critical components is indispensable for innovation.
1. High Temperatures
High temperatures are central to "deephot" analysis, representing a crucial parameter for investigating material properties under extreme conditions. This exploration focuses on how elevated temperatures influence material behavior, directly impacting the efficacy and outcomes of such analyses.
- Thermal Stability and Degradation
High temperatures frequently induce material degradation. Analysis at these levels helps understand how materials respond to stress and the onset of failure. This knowledge is critical in applications like aerospace engineering where components must withstand extreme heat. For instance, testing turbine blades at high temperatures reveals their endurance limit and necessitates optimization for increased efficiency and longevity. Understanding the material's thermal stability directly contributes to "deephot" analysis, allowing for the identification of suitable materials for specific applications.
- Phase Transitions and Transformations
Material behavior often changes dramatically at specific high-temperature thresholds. Understanding these phase transitions is vital for predicting a material's properties and structural integrity. For example, determining the crystalline structure and bonding changes in metals at high temperatures can help develop alloys with unique functionalities. This deep understanding is essential within the context of "deephot" studies.
- Reaction Kinetics and Mechanisms
High temperatures frequently accelerate chemical reactions. Studying the rates and mechanisms of reactions at elevated temperatures gives insight into the material's reactivity and helps optimize processes such as those in combustion engines. Analysis at elevated temperatures underpins the understanding of reaction kinetics needed for a comprehensive "deephot" approach.
- Mechanical Properties at High Temperatures
Material strength, elasticity, and ductility can alter significantly with increasing temperature. High-temperature testing is integral to determining a material's performance under extreme conditions, critical for design applications that require materials to withstand these conditions. This knowledge directly influences decisions in "deephot" research, particularly in choosing suitable materials that maintain desired performance at high operational temperatures.
In summary, understanding material responses to high temperatures is intrinsically linked to "deephot" analysis. It provides insight into the material's suitability for a given application under demanding thermal conditions, allowing for the prediction of behavior and the identification of optimal design solutions.
2. Material Properties
Material properties are fundamental to in-depth thermal analysis. They dictate a material's response to high temperatures, forming the bedrock of understanding for "deephot" studies. The relationship is causative; material properties determine the outcome of thermal experiments, influencing behavior under extreme conditions. High-temperature strength, thermal conductivity, and resistance to degradation are examples of properties critical for evaluating material performance within the framework of in-depth thermal analysis.
Consider the design of aircraft engines. Materials must exhibit exceptional high-temperature strength to withstand intense heat generated by combustion. Precise measurements of these properties during "deephot" analysis ensure the engine's longevity and safety. Similarly, in the development of advanced energy storage technologies, understanding the thermal stability of materials is paramount. "Deephot" investigations of electrochemical storage devices rigorously test materials' ability to resist degradation at operational temperatures, crucial for optimizing performance and extending cycle life. The thermal expansion and contraction characteristics of a material are equally important parameters, influencing the structural integrity of components under thermal stress, as seen in the design of spacecraft heat shields. "Deephot" methods determine the material's capacity to withstand the immense heat generated during atmospheric entry. In every case, material properties are not merely components; they are the very essence of "deephot" analyses, driving the design and development process.
In conclusion, material properties are integral to "deephot" analysis, defining a material's performance under extreme thermal conditions. The correlation is undeniableunderstanding these properties is crucial for predicting behavior, optimizing design, and ultimately improving various technologies. By thoroughly examining material responses to high temperatures through "deephot" analysis, researchers gain the knowledge needed to develop advanced, resilient, and efficient materials for a diverse array of applications.
3. Reaction Kinetics
Reaction kinetics, the study of the rates and mechanisms of chemical reactions, plays a critical role in "deephot" analysis. High temperatures significantly influence reaction rates, making a thorough understanding of kinetics essential for accurate predictions within "deephot" experiments. Elevated temperatures accelerate reaction speeds, often dramatically, which necessitates sophisticated analyses to fully understand the interplay between temperature, reaction rate, and the resulting material changes. For instance, in combustion engines, controlling reaction kinetics at high temperatures is paramount to efficient fuel combustion. Similarly, in the design of catalysts used in industrial processes, the kinetics of reactions at elevated temperatures dictate catalyst efficiency and lifespan.
The importance of reaction kinetics within the context of "deephot" analysis extends beyond simply understanding rate accelerations. Detailed kinetic analysis provides insights into the reaction mechanisms at play, unveiling details that impact product yields, selectivity, and the nature of any side reactions. In metallurgy, understanding the kinetics of oxidation reactions at high temperatures is crucial for the production of high-quality alloys. High-temperature studies also help determine the activation energy for reactions, providing valuable information that allows for the optimization of processes and selection of optimal materials for specific applications. Precise understanding of these kinetics is crucial for successful "deephot" investigations, enabling researchers to effectively predict and control the complex interactions involved in high-temperature environments.
In conclusion, reaction kinetics is an integral component of "deephot" analysis, providing a vital understanding of the dynamic relationships between temperature, rate, and mechanism. Precise control and prediction of chemical reactions at elevated temperatures are essential for optimizing processes, developing advanced materials, and gaining deeper insights into the intricacies of high-temperature phenomena. The precise quantitative understanding of these interactions within "deephot" analyses remains crucial for the development of new technologies and advancements in various fields.
4. Phase Transformations
Phase transformations, shifts in a material's physical state (e.g., solid to liquid, solid to gas), are inextricably linked to "deephot" analysis. Elevated temperatures frequently trigger these transformations, making their study essential within this framework. Understanding how materials behave during transitions under high temperatures directly impacts the success of "deephot" investigations. Changes in structure and properties during these transformations are crucial for anticipating the material's overall response and performance under extreme thermal conditions.
Consider the development of advanced alloys. Precise control over phase transformations during the cooling process is vital in achieving desired mechanical properties. High-temperature experiments are essential in determining the conditions for creating specific crystalline structures and associated characteristics. For instance, understanding the phase transformations in titanium alloys at high temperatures is vital for creating stronger, lighter materials suitable for aerospace applications. Predicting these transformations accurately during "deephot" investigations is essential for material design and optimization. Similarly, in the production of semiconductors, the control of phase transitions during crystal growth at elevated temperatures directly affects the electronic properties of the final product, underlining the critical role of phase transformations within "deephot" analysis. The formation of new crystalline phases and their subsequent stability in these materials are direct outputs of the "deephot" analysis process.
In summary, phase transformations are critical components of "deephot" investigations. Precise control over these transformations at high temperatures is essential for creating materials with desired properties. Understanding the temperature-dependent phase diagrams and the resulting structural changes in materials enables sophisticated control over phase transformations and leads to innovative material development. A deep understanding of phase transformations is, therefore, crucial for many aspects of "deephot" analysis, impacting the development of more advanced and resilient technologies across diverse fields, from metallurgy to materials science to semiconductor engineering.
5. Thermal Conductivity
Thermal conductivity, a material's capacity to transfer heat, is a critical component within "deephot" analysis. High temperatures often necessitate accurate measurements of thermal conductivity to predict and control heat transfer within systems. The relationship is causal; thermal conductivity directly influences a material's response to thermal stresses and heat fluxes at elevated temperatures. Precise measurement and understanding of thermal conductivity at high temperatures are essential for various applications, including designing advanced heat exchangers, optimizing thermal management in electronic devices, and predicting the behavior of materials subjected to intense heat sources.
Accurate thermal conductivity values at elevated temperatures are vital in numerous engineering applications. Consider the design of high-performance engines. High thermal conductivity materials are necessary for efficient heat dissipation from engine components. Without precise conductivity data at high operating temperatures, the engine design could lead to overheating, reduced efficiency, and potential component failure. Similarly, in the development of advanced energy storage systems, understanding the thermal conductivity of electrode materials is crucial for optimizing charge-discharge rates and preventing overheating during operation. The ability to precisely predict the thermal response of materials under high temperatures provided by detailed thermal conductivity measurements is vital for minimizing heat-related issues within these critical components. Furthermore, in high-temperature furnaces, understanding the thermal conductivity of materials within the furnace walls and surrounding structures allows for optimal design and prevents overheating, ensuring longevity and operational safety.
In summary, thermal conductivity is a fundamental property influencing a material's behavior at high temperatures. Accurate measurements and modeling of this property are essential for "deephot" analysis in numerous fields. This understanding enables the development of more efficient and durable technologies, reducing the risk of material failures and maximizing performance across a range of applications, from energy systems to aerospace engineering.
6. Mechanical Strength
Mechanical strength, the capacity of a material to withstand stress without failure, is intrinsically linked to "deephot" analysis. High temperatures significantly alter material properties, impacting mechanical strength. Understanding this relationship is paramount for predicting and mitigating failures in high-temperature environments. Elevated temperatures often reduce a material's strength, leading to decreased resistance against deformation or fracture. Consequently, accurate evaluation of mechanical strength at elevated temperatures is essential for critical applications.
Consider the design of jet engine components. Turbine blades, subjected to intense heat and stress during operation, require exceptional mechanical strength at high temperatures. "Deephot" analysis, focusing on material behavior at these extreme conditions, is critical for determining the optimal material selection and component design. Failing to account for the temperature-dependent reduction in strength could lead to catastrophic failure. Similarly, in nuclear reactor design, understanding the mechanical strength of structural components at elevated temperatures is critical for safety. The material must maintain its structural integrity under the immense pressure and heat, preventing potential meltdowns or other catastrophic events. Accurate "deephot" analysis ensures that components can withstand these stresses, upholding safety standards in these high-stakes environments. Further, in spacecraft heat shields, the ability of materials to resist deformation and fracture at high temperatures is vital for protecting the spacecraft's internal components during re-entry.
In conclusion, mechanical strength at elevated temperatures is a pivotal consideration within "deephot" analysis. A comprehensive understanding of how temperature affects material strength is crucial for ensuring the reliability and safety of various engineering applications. This knowledge enables the selection of appropriate materials, design optimization, and predictive modeling for high-temperature environments, fostering the development of more robust and resilient technologies in diverse sectors.
7. Advanced Materials
Advanced materials play a critical role in "deephot" analysis. Their unique properties, particularly at elevated temperatures, directly influence the outcomes of in-depth thermal studies. Developing materials capable of withstanding extreme heat and maintaining structural integrity is fundamental to many high-tech applications, making the study of these materials essential for advancing the scope of "deephot" investigations.
- High-Temperature Strength and Stability
Advanced materials often exhibit exceptional strength and stability at high temperatures. This characteristic is essential for components operating in extreme thermal environments. Examples include high-temperature alloys utilized in aerospace engines, enabling sustained operation under intense heat and stress. The study of these materials under high temperatures, as part of "deephot" analysis, is vital for predicting performance limits and identifying optimal designs for longevity and safety in high-temperature applications.
- Thermal Conductivity and Management
Certain advanced materials possess exceptional thermal conductivity. These materials are crucial for efficient heat dissipation in high-power applications. For instance, heat sinks in electronic devices utilize materials with high thermal conductivity to manage heat buildup and maintain optimal performance. Examining these materials within the framework of "deephot" analysis allows for the optimization of thermal management systems and the design of more effective heat dissipation strategies.
- Resistance to Oxidation and Degradation
Many advanced materials exhibit superior resistance to oxidation and degradation at high temperatures. This characteristic is crucial for components exposed to corrosive environments or extreme thermal cycles. Coatings and alloys designed for this purpose are used in industrial furnaces and high-temperature reactors. Assessing these materials' performance in "deephot" tests provides crucial data for the selection of appropriate materials for specific applications requiring extended operation in aggressive thermal environments.
- Enhanced Mechanical Properties under Extreme Conditions
Advanced materials frequently demonstrate enhanced mechanical properties even at high temperatures, making them suitable for applications under extreme thermal stress. These materials are essential for structures subjected to severe thermal cycles, such as those found in rocket engines and hypersonic vehicles. Analysis of these properties within the "deephot" framework helps predict material behavior under various thermal load conditions, fostering safer and more efficient design strategies.
In conclusion, advanced materials are integral to "deephot" investigations. Their unique properties, particularly their ability to withstand high temperatures and maintain desired performance, are vital for numerous high-tech applications. The development and testing of these materials under controlled thermal conditions are central to the success of "deephot" analysis, driving innovation and advancement in various sectors.
Frequently Asked Questions about In-Depth Thermal Analysis
This section addresses common inquiries regarding in-depth thermal analysis, often referred to as "deephot" studies. These questions cover crucial aspects of methodology, application, and interpretation of results.
Question 1: What are the key objectives of "deephot" analysis?
The primary objectives of in-depth thermal analysis encompass understanding material behavior under extreme thermal conditions. This includes evaluating thermal stability, determining phase transformations, assessing reaction kinetics at elevated temperatures, and precisely measuring thermal conductivity. The goal is to predict how materials will perform under challenging thermal stresses.
Question 2: How does "deephot" analysis differ from standard thermal analysis techniques?
In-depth thermal analysis typically involves more rigorous and comprehensive testing protocols. It often employs advanced instrumentation and specialized experimental setups. While standard thermal analysis methods can provide valuable baseline data, "deephot" analysis focuses on pushing materials to extreme limits, characterizing their performance at much higher temperatures and for longer durations, and achieving a more fundamental understanding of underlying mechanisms.
Question 3: What are the applications of "deephot" analysis in various industries?
Applications span a multitude of industries. In materials science, it allows for the development of advanced alloys with specific high-temperature properties. In aerospace engineering, it aids in designing more efficient and durable engine components capable of withstanding intense heat. Furthermore, it's integral for research in fields like nuclear engineering, where understanding the behavior of materials under extreme temperatures is crucial for safety and performance. High-temperature catalytic processes also benefit from "deephot" analysis, enabling the optimization of reaction kinetics and catalyst design.
Question 4: What are the limitations of current "deephot" analysis methods?
Limitations often arise from the inherent challenges of studying materials at high temperatures. Maintaining precise temperature control, ensuring sample integrity, and accurately measuring thermal properties under these demanding conditions can be complex. The very high temperatures sometimes used in experimentation can introduce errors and create limitations in certain analytical methods. In addition, the cost of specialized equipment and the complexity of data interpretation can pose limitations as well.
Question 5: What future directions are anticipated for "deephot" analysis?
Future trends encompass enhanced instrumentation for more precise measurements, improved modeling and simulation techniques to better interpret complex data sets, and innovative materials capable of withstanding extreme thermal stresses. Furthermore, integration of advanced experimental methods with computational modeling will likely lead to more accurate predictions and a deeper understanding of material behavior in extreme environments.
In conclusion, "deephot" analysis is a vital tool for understanding and characterizing material behavior at high temperatures. By addressing these common inquiries, this FAQ aims to facilitate a better understanding of this crucial aspect of materials science and engineering. The next section will delve into specific methodologies employed in "deephot" studies.
Conclusion
In-depth thermal analysis, often referred to as "deephot," provides a crucial framework for comprehending material behavior under extreme thermal conditions. Key aspects of this analysis, including high-temperature material properties, reaction kinetics, phase transformations, thermal conductivity, mechanical strength, and the development of advanced materials, were explored. The study of these elements under elevated temperatures is critical for numerous applications, from designing aerospace components to optimizing industrial processes and advancing energy technologies. Understanding the intricate relationship between temperature and material characteristics is fundamental to progress across diverse scientific and engineering domains.
The future of "deephot" analysis hinges on advancements in instrumentation, modeling techniques, and the development of novel materials. Further research will undoubtedly lead to improved predictive capabilities, enabling the creation of more efficient, robust, and sustainable technologies. Precise characterization of materials under extreme thermal conditions remains a cornerstone for technological innovation and critical to tackling contemporary challenges in fields ranging from aerospace to energy production. Continued exploration and refinement of "deephot" analysis methods are essential for progress in these sectors.
Article Recommendations
- Joe Burrows Wifes Battle With Cancer A Journey Of Love And Resilience
- Emily Compagno Wedding A Celebration Of Love And Elegance
- Angie Hermon The Rising Star In The Entertainment Industry


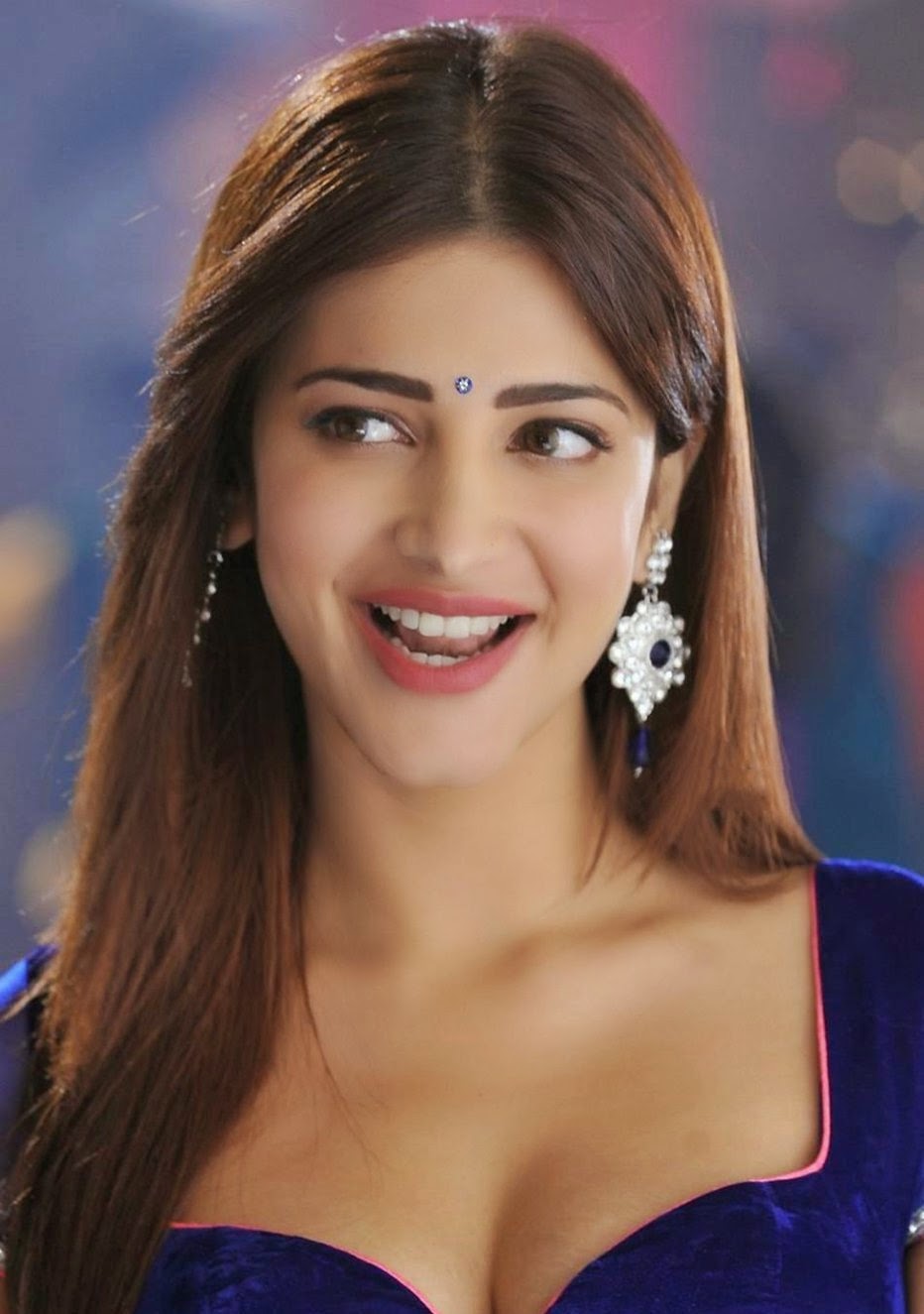